Instrument: Lightning Instrument Package (LIP)
The Lightning Instrument Package (LIP) is an airborne electric field detection system that can be flown onboard a variety of aircraft including NASA’s ER-2 high-altitude research aircraft, DC-8 aircraft, and Global Hawk Unmanned Aerial System (UAS). LIP measures the three-dimensional components of the electric field (i.e., Ex, Ey, Ez) in the thunderstorm environment. To do this, LIP uses a network of 5 to 8 rotating-vane style electric field mills and a dual-tube conductivity probe, installed at different locations on the aircraft. The electric field mills are compact sensors, each weighing less than 10 lbs. The main components of each field mill are the stationary sensor plates and the 2-blade rotor (Figure 1). When the rotor rotates, it alternately covers and exposes the sensor plates to the surrounding, environmental electric field; collecting 50 samples per second. The sensor plates are being “charged” by the electric field when they are exposed and are being “discharged” when covered. The alternating charge on the sensor plates creates a signal whose amplitude is proportional to the vector components of the electric field. The conductivity probe consists of a pair of capacitor type sensors. The probe measures air conductivity at aircraft flight level by measuring positive and negative ions simultaneously throughout the flight.
Measures the three-dimensional components of the electric field (i.e. Ex, Ey, Ez). Each of the LIP field mills incorporate self-calibration capabilities that reduce the time required to obtain a full aircraft calibration. In addition, the electric field signals from each field mill are digitized and transmitted as a digital data stream, reducing signal noise and simplifying aircraft integration.
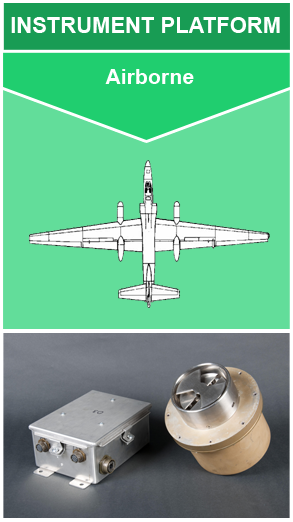
SPATIAL RESOLUTION | DYNAMIC RANGE | ACCURACY | NOISE | SAMPLING DURATION |
---|---|---|---|---|
Point
(a single vector is generated by the horizontal and vertical components of the electric field)
|
Sensitive channel:
± 1.9 V m-1 - 64 kV m-1
Insensitive channel:
± 34 V m-1 - 1.1 MV m-1
|
± 2.5% |
Zero field error: ≤ ±25 V m-1
Field uncertainty: ≤ ±1.9 V m-1
|
50 samples per second |
Bateman, M.G., Stewart, M.F., Podgorny, S.J., Christian, H.J., Mach, D.M., Blakeslee, R.J., ... Daskar D. (2007). A Low-Noise, Microprocessor-Controlled, Internally Digitizing Rotating-Vane Electric Field Mill for Airborne Platforms. Journal of Atmospheric and Oceanic Technology, 24, 1245–1255. https://doi.org/10.1175/JTECH2039.1
Hood, R.E., Cecil, D.J., LaFontaine, F.J., Blakeslee, R.J., Mach, D.M., Heymsfield, G.M., ... Goodman, M. (2006). Classification of Tropical Oceanic Precipitation using High-Altitude Aircraft Microwave and Electric Field Measurements. Journal of the Atmospheric Sciences, 63, 218–233. https://doi.org/10.1175/JAS3606.1
Koshak, W. J., Mach, D. M., Christian, H. J., Stewart, M. F., & Bateman, M. G. (2006). Retrieving storm electric fields from aircraft field mill data. Part II: Applications. Journal of Atmospheric and Oceanic Technology, 23, 1302–1322. https://doi.org/10.1175/JTECH1918.1
Mach, D. M., & Koshak, W. J. (2007). General matrix inversion technique for the calibration of electric field sensor arrays on aircraft platforms. Journal of Atmospheric and Oceanic Technology, 24, 1576-1587. https://doi.org/10.1175/JTECH2080.1
Marshall, T. C., Stolzenburg, M., Maggio, C. R., Coleman, L. M., Krehbiel, P. R., Hamlin, T., ... Rison, W. (2005). Observed electric fields associated with lightning initiation. Geophysical Research Letters, 32, L03813. https://doi.org/10.1029/2004GL021802